In the year 2018, Lise Meitner was honored by the University of Vienna. She was a physicist, who should have been awarded several times with the Nobel Price, but never got it. There are many press articles that write about her life, but very few concerned with the physics that was so fascinating for her.
About her Life
Elise (Lise) Meitner was born on the 7th of November 1878 to an upper middle class Jewish couple in Vienna. Her father, Phillipp Meitner was one of the first Jewish lawyers in Austria. From her childhood, young Lise was fascinated by the aura of science and mathematics, but the restrictions imposed on women to pursue studies in public institutions of higher educations in that time forced her to train herself with private education in physics and successfully complete an external matura examination at the Akademisches Gymnasium. She then entered the University of Vienna and her enthusiasm for physics drove her to the lectures of Ludwig Boltzmann, whose humor was contagious. It is said that ‘’Boltzmann gave her the vision of physics as a battle for ultimate truth, a vision she never lost.’’ Boltzmann's assistant Stefan Meyer was the one to introduce Lise to the exciting new field of radioactivity. Thus, after her dissertation with Franz Exner, where she studied heat conduction in inhomogeneous media, she decided to change her studies to radioactivity. At that time she wrote her first article about the absorption of alpha and beta radiation.
Shortly afterwards she met Max Planck in Vienna. Thanks to Planck, Meitner applied for a job in Berlin. She got a job in the laboratory of the experimental physicist Heinrich Rubens and registered for the physics colloquium, where she made the acquaintance of Otto Hahn, an exceptional radiochemist who was considered an outsider in Berlin. A great scientific friendship was born. In the early 1910s, Meitner and Hahn conducted joint research on the absorption and behavior of alpha and beta radiation in magnetic fields.
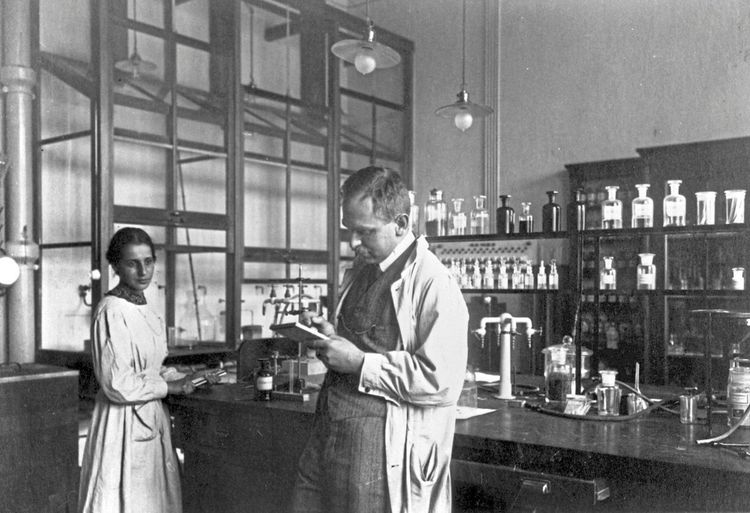
At the end of World War I, she became professor of the physics department at the Kaiser Wilhelm Institute of Chemistry, where she pursued her own research. In 1935 she wrote a monograph together with the theorist Max Delbrück entitled "The Structure of Atomic Nuclei". In 1938 she came together with Otto Hahn again and they discovered together the nuclear fission of uranium – a discovery that changed human history forever. But the Nazi regime changed everything. In July 1938, with the help of Hahn and Dirk Coster, she had to flee to Stockholm, where nine years later she became head of the nuclear physics department at the Royal Institute of Technology in Stockholm. She died on the 27th of October 1968 in Cambridge, where she spent the last eight years of her life working with her nephew Otto Frisch.
Nuclear physics in short
Matter is formed of atoms that are composed of a nuclei and electrons. The nucleus, in turn, consists of the positively charged particles called protons (Z) and the electrically neutral components called the neutrons (N). The typical size of an atom is about 0.1 nm (0.00000001 cm) and the nucleus is about 10000 times smaller. It is common knowledge that opposite attract themselves, so that the negative electrons are attracted to the positive protons in the nucleus. This attractive force between negative and positive charged particles is the Coulomb force. However, the situation becomes different in the nucleus where only the positively charged protons reside, because according to the good old law that states that equal charges repel each other, would make it difficult for the nucleus to survive. But it is here where the extremely small dimension of the nucleus comes into play and the strong attractive nuclear force dominates over the repulsive Coulomb force leading to a stable nucleus and in turn a stable atom.
Atoms must be charge neutral, this means that they should have the same number of electrons and protons. A nucleus with the same number of protons but different number of neutrons are called isotopes. The stable atoms in nature are usually those whose nuclei have an even-even amount of protons and neutrons. However, this is not always the case. Radioactivity can be explained in a very simple picture by the instability of the nucleus, which can be regarded as a charge imbalance. So in order to reach a stable state, the nucleus emits radiation. During this process, called nuclear decay or radioactive decay, the number of protons, neutrons or electrons in the nucleus changes, and the nucleus either ends up in a stable state or can decay further.
This emitted radiation can be either alpha-radiation, beta-radiation or gamma-radiation. The properties of these radiations, also known as radioactive particles, are very different. It was partly the work by Lise Meitner and Otto Hahn on their behavior in magnetic fields that gave more information on their nature. They placed a piece of Thorium, an element known to be unstable, in a rod and observed the deflection of beta-radiation in a magnetic field at a fluorescent screen. When electrons move in a magnetic field, they change their trajectory from straight to circular or helical. In the same manner, they observed that the beta-rays bended in a direction that indicated that they were negatively charged particles. Interestingly, the beta-radiation didn’t always reach the same spot on the screen, indicating that beta-radiation has a wide number of energy values.
Alpha-radiation are stable Helium nuclei, consisting of two protons and two neutrons, and is mono-energetic, this means it has one single energy value of 5000000 eV (5 MeV). Beta-radiation consists mainly of electrons and its energies lie between 1-3 MeV. Gamma-radiation, on the other hand, is unaffected by magnetic fields, leading to the conclusion that it consists of uncharged particles. Indeed, gamma-radiation consists of photons, this means light-particles. The most known gamma-radiation are x-rays.
Based on her studies on the absorption and nature of alpha- and beta-radiation, Meitner was able to foretell approximately how an eventual nuclear reaction would proceed when bombarding an unstable element with alpha- or beta-particles.
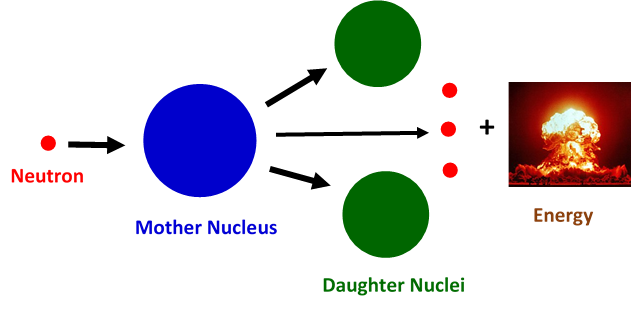
Nuclear fission: the phenomena that changed the course of humanity
The story of nuclear fission probably begun with the discovery of neutron by James Chadwick in 1932. Following this discovery, Enrico Fermi and his co-workers in Rome studied the results of bombarding uranium with neutrons, where they demonstrated the existence of new radioactive elements produced. However, there was a lack of understanding of the actual physical mechanism that governed this process. There is a very simple picture to understand nuclear fission: one has to consider the nucleus as a spherical drop of liquid. An incident neutron can lead to deformations of the original liquid drop and change its shape. The liquid drop can elongate and create two lobes that will separate, causing a split or fission of the initial nuclear drop into two droplets. In a similar way, during nuclear fission a mother nucleus transforms into two or more daughter nuclei.
In 1938, Hahn reported to Meitner that when bombarding the 238-Uranium isotope (N=146 and Z=92) with neutrons nine beta-decays were produced, leaving at the end the formation of barium (Z=56). This was an unexpected result and Otto Frisch, her nephew who had joined his aunt research, named it nuclear fission, after the similar phenomenon observed in cells. Meitner, together with Frisch, concluded that apart from barium, the uranium had produced Xenon (Z=36) with huge amounts of energy needed to do so. And where did this energy come from? Here, thanks to Albert Einstein’s famous mass-energy equivalence formula of E = mc², the amount of energy that could be generated in a single fission event could be calculated. The quantity m is the difference of the nuclear masses of the daughters and the mother nuclei and c denotes the speed of light. Now, since the value of c is known to be 300 000 km/s, whatever small the mass difference might be, the energy yield in a fission event is enormous – much greater than chemical combustion ever yielded.
The news of the discovery of nuclear fission soon reached the other side of the Atlantic, as in Europe loomed the dark shadow of the World War II. While nuclear fission could have served as a source of sustainable energy, soon it was exploited to build the first nuclear weapon through the Manhattan project. When controlled, nuclear fission, could give us useful energy, but in an uncontrolled chain reaction, even one kilogram of uranium (U-235) results in a yield of 17.5 kilotons of TNT (trinitrotoluene). And so the gift of science and the honest conviction of scientists were lost at the hands of politics.
Her legacy
Meitner always stayed in contact with Hahn and had to watch her research partner being honored with the Nobel Prize in Chemistry in 1945 for their joint work on nuclear fission. Nevertheless, she never let herself down. Lise Meitner was sure of her contribution. She knew what she had achieved, even if it was only recognized much later. She was very critical about the use of nuclear fission in weapons, but she loved her work: "I love physics, it's hard to imagine my life without it." Lise Meitner was a self-confident woman, whose enthusiasm for physics is contagious and should serve as an inspiration, not only to young women, but to every child who wants to explore nature through the tools of science and mathematics and to bring a positive change to humanity as a whole. (Andrea Navarro-Quezada, Rajdeep Adhikari, 8.3.2020)
To the german version of this blogpost
On the subject
More posts from the bloggers